The Lowdown on Neuronal Tuneups
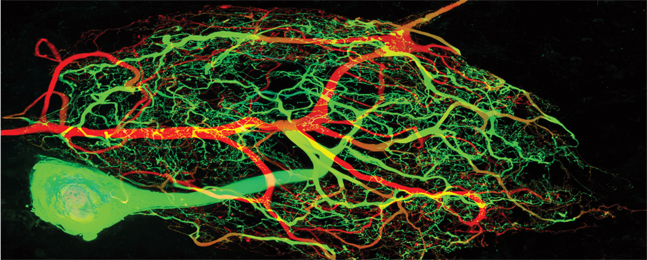
Marie Goeritz/Ted Brookings
IN USE: An individual neuron can rebuild itself without disturbing homeostasis across the nervous system.
by Leah Burrows
When your car needs a new spark plug, you take it to a shop, where it sits, out of commission, until it’s repaired. But what if your car could replace its own spark plug while speeding down the Mass Pike?
The human nervous system is able to do the equivalent — rebuild itself continually while maintaining full function.
Although a neuron lives for many years, the proteins and molecules that are its components are continually being replaced. How the rebuilding happens without affecting our ability to think, remember, learn or otherwise experience the world is one of neuroscience’s grand questions.
Eve Marder ’69, the Victor and Gwendolyn Beinfield Professor of Neuroscience, is working toward finding an answer. As the May 21 issue of the neuroscience journal Neuron reports, Marder’s lab has built a new theoretical model to understand how cells monitor and self-regulate their properties amid continual turnover of cellular components.
Ion channels, the molecular gates on cell surfaces, determine neuronal properties needed to regulate everything from the size and speed of limb movement to the processing of sensory information; different combinations of ion-channel types are found in each kind of neuron. Receptors are molecular “microphones” that enable neurons to communicate with one another.
Because ion channels and receptors are constantly turning over, cells need to regulate the rate at which they are replaced in a way that avoids disrupting normal nervous system function. Scientists have considered the idea of a “factory” or “default” setting for the numbers of ion channels and receptors in each neuron. But because a neuron’s environment changes so much over the course of its life, this idea seems implausible.
So, if there is no factory setting, neurons need an internal gauge to monitor electrical activity and adjust ion channel expression accordingly, the Marder team asserts. Since a single neuron is always part of a larger circuit, it also needs to do this while maintaining homeostasis across the nervous system.
The Marder lab built a new theoretical model of ion channel regulation based on the concept of an internal monitoring system. The team — postdoctoral fellow Timothy O’Leary; lab technician Alex Williams; Alessio Franci, of the University of Liège in Belgium; and Marder — discovered that cells don’t need to measure every detail of activity to keep the system functioning. In fact, too much detail can derail the process.
“Certain target properties can contradict each other,” O’Leary says. “You would not set your air conditioning to 64 degrees and your heat to 77 degrees. One might win over the other, but they would be continually fighting each other and you would end up paying a big energy bill.”
The team also learned that cells can have similar properties but different ion channel expression rates — like cellular homophones, they sound alike but look very different.
According to the model, the very internal monitoring system designed to control runaway electrical activity can also lead to neuronal hyperexcitability, which underlies seizures. Even if set points are maintained in single neurons, the system’s overall homeostasis can be lost.
The study represents an important advance in understanding the most complex machinery ever built — the human brain. And it may lead to entirely different therapeutic strategies for treating diseases, O’Leary says.
“To understand and cure some diseases, we need to pick apart and understand how biological systems control their internal properties when they are in a normal healthy state,” he says. “This model could help researchers do that.”