Breakthrough Research May Help Reduce Viral Load in Infections
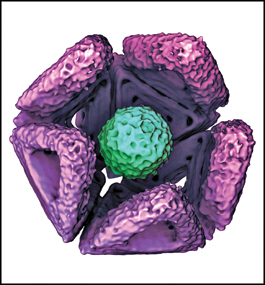
Chris Sigl / Technical University of Munich
LIKE AN INSECT IN A PITCHER PLANT: A cryo-electron microphotograph of a self-assembled icosahedral shell, made from DNA fragments, that has trapped a hepatitis B virus particle. Antibodies on the shell’s interior keep the virus bound inside.
by Richard Collins
Brandeis researchers knew they had a major scientific accomplishment on their hands when, for the first time, they and their collaborators at the Technical University of Munich were able to program bits of DNA strands to fold up and self-assemble into predetermined complex geometric shapes.
Then, in the wake of the global coronavirus pandemic, came a moonshot idea: Could those tiny geometric structures be used as an antiviral agent? To everyone’s amazement, they could.
“It’s beyond my wildest dreams that this would work,” says physicist Seth Fraden, PhD’87, director of Brandeis’ Materials Research Science and Engineering Center. “It’s just so exciting. I can’t sleep at night thinking about what we can do with this stuff.”
Fraden, along with Michael Hagan, a physics and quantitative biology professor at Brandeis, detailed the accomplishment in a paper published in Nature Materials. The trans-Atlantic research team used microscopic triangular building blocks to create shells capable of capturing viruses and neutralizing them. Although the breakthrough isn’t ready for patient trials yet, it opens a potential new avenue for treating deadly pathogens, administering drugs or delivering gene therapies.
“This is a positive test of the theory and takes a big step toward eventually having these applications,” says Hagan.
In a basic sense, the achievement amounts to humans figuring out how to do something viruses — which are very primitive biological structures — have been doing for a billion years, Fraden says. Viruses can contort and assemble themselves into highly efficient structures that can wreak havoc on host bodies. Figuring out from an engineering standpoint what makes this behavior possible is something the Brandeis team has been working on for years.
“We asked, ‘OK, if viruses use these very efficient engineering principles to assemble themselves, why can’t we as material scientists make nanostructures based on the same engineering principles the viruses use?’” Fraden says.
“You don’t really understand something unless you’re able to take those design principles and from scratch build a system based on those principles and see if it works,” Hagen says.
Fraden’s team, who had earlier partnered with Hendrik Dietz at the Technical University of Munich on other nanotechnology projects, proposed exploring the creation of a programmable artificial-shell platform in 2016.
The Brandeis team had previously come up with the theoretical idea of using triangles made up of DNA to efficiently construct various geometric shapes — think Buckminster Fuller’s famous geodesic dome. The trick was demonstrating it is actually possible to program DNA to fold in specific ways, and to have the folded DNA bits find one another and latch together like a self-assembling Lego structure. The work is built on principles elucidated by Donald Caspar, professor emeritus of biology, who coined the term “structural biology.” In the 1970s, Caspar established a Brandeis lab that studied viruses’ molecular structure.
Fraden says Dietz’s Technical University of Munich team are world leaders in the nanobiotechnology field known as “DNA origami,” or designing DNA that can fold to create complex 3D forms.
“Combining their expertise in origami with our expertise in self-assembly led to incredibly rapid advances,” Hagen says.
Then COVID-19 struck, and the Munich researchers realized the potential value of expanding the experiment to see if shells that effectively place viruses in quarantine could be constructed. Researchers programmed the DNA to create icosahedron-shaped capsids, with apertures just wide enough to engulf a virus — in this case, a hepatitis B or adeno-associated virus.
Much like an insect entering a pitcher plant, once inside the shell the virus can’t escape. Antibodies applied to the shell’s interior kept the viruses bound inside. The shell also acted as a protective bumper, preventing the virus’s spike proteins from latching onto a host.
“It worked especially well, way beyond what we imagined possible,” Fraden says.
Though the innovation carries many possible implications for nanotechnologies and material sciences, it’s the biopharma side that really has researchers jazzed. Vaccinations trigger an immune system response in patients before they contract a virus, and some antivirals interrupt replication during active infections. But there currently is no effective treatment that reduces viral load. With some viruses, such as COVID-19, a high viral load can increase both transmission risk and the severity of the illness.
“If you have a heavy load of virus in your body, this is a potential platform to effectively sweep your body clean of the virus,” Fraden says.