A new twist on surface tension
On a mission to manipulate microscale structures of materials, researchers engineer new methods of controlling surface tension
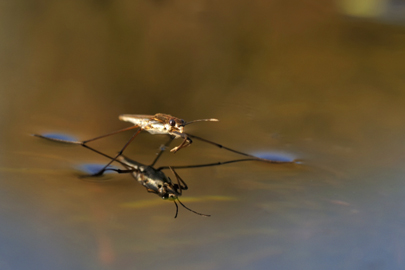
Surface tension allows skimmer to stand on water
Ever wonder what trees, water skimmers and laundry detergent have in common? It turns out that the physical concept of surface tension is essential to their function.
The idea behind surface tension is that in a mixture of two molecular components -- let’s call them component "A" and component "B" -- the "A"s would really like to stick with their fellow "A"s and don’t want to be next to any "B"s, similar to boys and girls at a sixth-grade dance.
If you pour oil on a cup of water, the oil will quickly separate out to the top of the mixture to minimize the contact area between the two components. The higher the magnitude of the surface tension, the more the "A"s cannot stand the "B"s. Surface tension is important in many natural processes, including allowing trees to carry nutrients from the roots out to the branches and water skimmers to walk on the surface of water.
Mixing it up: varying the surface tension
The interface between hydrophobic (oily) and hydrophilic (watery) components has very high interfacial tension, or surface tension. The magnitude of surface tension can be adjusted by adding amphiphilic molecules, ones that contain both hydrophilic and hydrophobic components, like soaps. These amphiphilic molecules prefer to be at the interface between the two components, and effectively lower the interfacial tension, allowing the components to mix more easily. At sufficiently low interfacial tension, small droplets of oil begin to dissolve in capsules called “micelles” composed of the amphiphilic molecules. This is how detergent causes oily stains to dissolve in water.
In a recently published article in Nature, an interdisciplinary team of researchers at Brandeis headed by Zvonimir Dogic, and consisting of experimental, theoretical and computational physicists as well as biologists, has demonstrated a new way of controlling interfacial tension using a molecular property called “chirality,” or lack of mirror symmetry. Examples of chiral structures include human hands and a DNA double helix.
The study was performed on a model system of two-dimensional colloidal membranes, a flexible sheet composed of micrometer-sized rod-like particles. Because the rods are chiral, they tend to twist in a small angle with respect to neighboring rods. However, the geometry of the membrane prevents twisting in the structure’s interior; only along the perimeter can the rods twist. Increasing the strength of chirality, or twistiness of the rods, lowers the energy of the rods along the membrane’s edge, also lowering the interfacial tension.
By manipulating the microscopic shape, the team of researchers was able to create reversible transitions of a flat two-dimensional membrane to a one-dimensional twisted ribbon. Engineering this system that creates reversible transitions is part of an overall research mission to manipulate microscale structures of materials.
In the first movie, the twisted ribbons have much more interfacial area than the membranes, but are much “twistier” structures, and are therefore favored when the strength of chirality is relatively high.
Additionally, in the movie below, researchers illustrate how they can drive the same membrane-to-ribbon transition using optical tweezers, an instrument that uses laser light to grab objects and move them around.
This work presents a powerful new method to control the assembly of materials, the researchers found.
Mark Zakhary is a graduate student in The Martin Fisher School of Physics and a member of the Zvonimir Dogic Group.
Categories: Research, Science and Technology