A few mind-blowing inventions coming from Brandeis' Bioinspired MRSEC
With their new grant from the National Science Foundation, MRSEC scientists are seeking to build the next generation of nano-sized machines and materials.
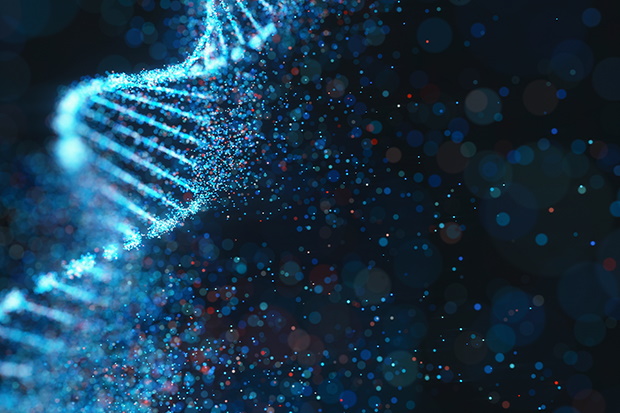
Self-healing wounds, a cure for viral infectious diseases and self-propelling liquids — these are a few of the big projects currently being developed at Brandeis' Bioinspired MRSEC.
These inventions may sound like the stuff of science fiction, but Brandeis Bioinspired MRSEC director and professor of physics Seth Fraden says, "The science is there. This is going to happen."MRSEC, which stands for Materials Research Science and Engineering Center, is a long-term, nationwide effort funded by the National Science Foundation to develop revolutionary new types of nano-sized materials and machines.
Last month, the NSF announced that Brandeis was one of only 11 universities to receive MRSEC funding. The $18 million, 6-year award will enable Fraden, his colleagues and the graduate students and undergraduates who work side-by-side with them to continue the cutting-edge research begun in 2008 when the center won its first NSF MRSEC grant.The Brandeis’ MRSEC aims to give human inventions powers and properties found in nature. By understanding such phenomena as the behavior of cellular proteins or the flight patterns of starlings, the center’s researchers hope they can imbue synthetic compounds with biologically-inspired abilities.
Here are descriptions of three cutting-edge MRSEC research endeavors and how scientists plan to turn them into reality.
SELF-HEALING WOUNDS
Archaea are some of the most mysterious organisms on the planet. They're single-celled like many bacteria, yet also share similar characteristics with human cells. Their hybrid nature has led them to be classified as a completely separate life form. Archaea have been found deep beneath the sea, in boiling geysers and some might even be able to survive on Mars.
They're of interest to MRSEC researchers in part because of the proteins in their outer cell membranes. These proteins are capable of arranging themselves in different ways, making archaeal cells wondrous shapeshifters, able to change into triangles, rods, circles and teardrops."They respond very fast and adapt into all different kinds of shapes and sizes," said assistant professor of biology Alexandre Bisson.
These transformations happen when there's a break in the cell envelope. Bisson is studying how these proteins could be harnessed to function in humans the way they do in archaea — to repair damaged cells. Specifically, Bisson is looking for ways to engineer and produce new proteins inspired by archaeal ones that could be injected into humans to heal tissues.VIRUS FIGHTING
One amazing power of biomolecules is their ability to "self-assemble," forming into elaborate three-dimensional structures on their own. Fraden and his collaborators would like to get synthesized DNA to fold up into the shape of a pitcher plant.
These plants are flute-shaped with an opening at the top. When flies enter the opening, they become stuck on the nectar inside and then digested.A pitcher plant made of DNA would work the same way. A virus would enter and then get caught, in this case on viral antibodies.
Using DNA, even if synthesized, has the advantage of introducing a compound into the human body that it is already used to.In addition, because DNA molecules self-assemble, they would arrange themselves into the shape of a pitcher plant autonomously. This has the advantage of not needing human intervention.
SELF-PROPELLING LIQUIDSProteins are extremely good at transporting cargo around our bodies. They carry oxygen, nutrients and sugar to cells.
One type of protein, kinesin, delivers vital cellular structures such as organelles and vesicles. Under a microscope, kinesin looks like a blob with a short pair of legs. It moves step by step along tracks called microtubules, hollow cylindrical rods found inside cells.Several years ago, Fraden and his collaborators bent a 3-foot long plastic tube into a closed circle, added some water and then sprinkled in some kinesin and microtubules. The microtubules organically arranged themselves into tracks and, just like they do in cells, the kinesin proteins then hopped aboard.
Next, Fraden and his team put in a molecule called adenosine triphosphate (ATP) which kinesin proteins use as energy to power their locomotion. As the kinesin began moving along the microtubules, they created a forward flow that also carried the water around the plastic tube.In other words, the liquid was self-propelling. It didn't need a human-made energy source such as a pump or motor to push it forward. It also moved without the aid of gravity. The plastic tube could be set flat on a table and the water still circulated.
Self-propelling fluids could be used in place of miniaturized conventional pumps. Fraden suggests a patch that people with diabetes could wear on their skin where synthesized kinesin and microtubules could be added to insulin.When the patch sensed it was needed, the kinesin and microtubules would get the insulin to start flowing into the wearer's body.
Categories: Research, Science and Technology