Liquids that can move on their own
Researchers at the Materials Research Science and Engineering Center (MRSEC) are harnessing the power of swirling cellular proteins to create self-propelling fluids.
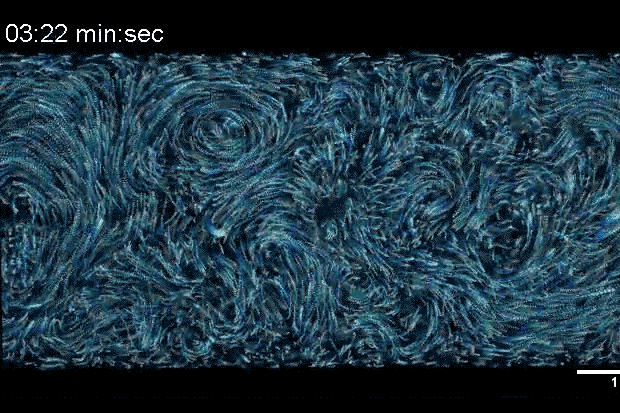
Two types of cellular proteins — kinesin and microtubules — interacting to create a vortex under the microscope.
Assistant professor of physics Guillaume Duclos calls it the "swirl."
It's made up of two types of cellular proteins -- kinesin and microtubules -- interacting to create a vortex under the microscope.
In the last year, researchers at Brandeis' Bioinspired Materials Research Science and Engineering Center (MRSEC) have taken significant steps toward understanding the swirl and controlling its flow.
When the swirl is placed in another liquid, it pushes it forward, creating what's called a "self-propelling" or "self-mixing" liquid. In other words, you don't need human or mechanical effort to get the liquid to move; it goes on its own.
One day, self-propelling liquids could be used to create a class of liquid drugs that are injected into the bloodstream and then autonomously flow toward a specific group of cells or organs. Because the swirl is made from components already in our cells, it wouldn’t be rejected by our bodies.
But to manufacture such “smart” medicines, we need to control the swirl's behavior, which means gaining a better understanding of the physics underlying its formation and movement. And that's exactly where the several recent papers by MRSEC scientists enter the picture.
What is the swirl?
Kinesin proteins transport molecules around cells. A kinesin molecule looks like a blob with a short pair of legs. It moves around along rails called microtubules, which make up the cells' internal scaffolding.
When a kinesin moves, it pushes one of its legs forward and the other backward. This causes the two microtubule rails on which the kinesin stands to move in opposite directions.
This motion of the microtubule rails pushes on the surrounding fluid to create a swirl.
A swirl in a container
Up until a few years ago, scientists had mainly studied the swirl in two dimensions, which essentially meant on the flat plane of a microscope slide.
But to make any practical use of the swirl, it will need to be placed inside a three-dimensional container. And scientists weren't sure how adding a dimension would affect the swirl's behavior.
In a 2017 paper in Science, Brandeis MRSEC director and professor of physics Seth Fraden and several colleagues reported on the relationship between the swirl's flow and the dimensions of the container in which it was placed.
Fraden and his team found that when the height and width of the container were roughly the same, the swirl moved continuously in one direction. The swirl carried the surrounding liquid to move forward, creating a self-propelling fluid. This is known as "coherent flow" or “circulation flow”
Incoherent flow, where the swirl moves side-to-side, forward and backward in a chaotic pattern, occurs when the height and width of the container are different.
Why this happened remained unclear until December, with the publication of another paper by professors of physics Michael Hagan, associate professor of physics Aparna Baskaran and several colleagues, in the journal Physical Review Letters.
Baskaran, Hagan and their collaborators worked out a theoretical model explaining how the container's height and width produce coherent flow. The model takes into account the incredibly complex mathematics underlying the behavior of the tens of millions of microtubules and kinesins in the swirl along with the force exerted by the container’s walls.
Spinning the swirl
Several years ago, it was discovered that the swirl responds to light. In a separate paper in Physical Review Letters in October, Hagan and Fraden showed how it would be theoretically possible to turn the swirl clockwise and counterclockwise using light.
Their research determined where the light needed to be placed in relation to the swirl and when the light needed to be turned on and off in order to change the swirl's direction.
The defective swirl
As the swirl swirls, what scientists call "defects" arise. These are essentially irregularities in the swirl's movement with "breakaway" groups of microtubules behaving differently than the microtubules in the rest of the swirl.
In addition, when the swirl is inside a container, the container’s walls push back on the microtubules. Since microtubules are fairly rigid, they don't bend, but instead, spring up vertically. This also causes irregularities in the swirl's motion.
If scientists want to control the swirl, they need a model that takes into account these defects and irregularities. The underlying mathematics is so complex, it's extremely difficult and time-consuming to calculate.
However, in a November paper in the journal Soft Matter, professor of computer science Pengyu Hong, Fraden and Hagan demonstrated how computers could learn purely from experimental data to predict the emergence and behavior of defects using deep learning techniques.
This is another important step towards understanding the swirl’s overall motion.
Categories: Research, Science and Technology