Coming Full Circle at the Large Hadron Collider
Brandeis’ high-energy physics group helped discover the Higgs boson, setting the stage for a major new wave of exploration.
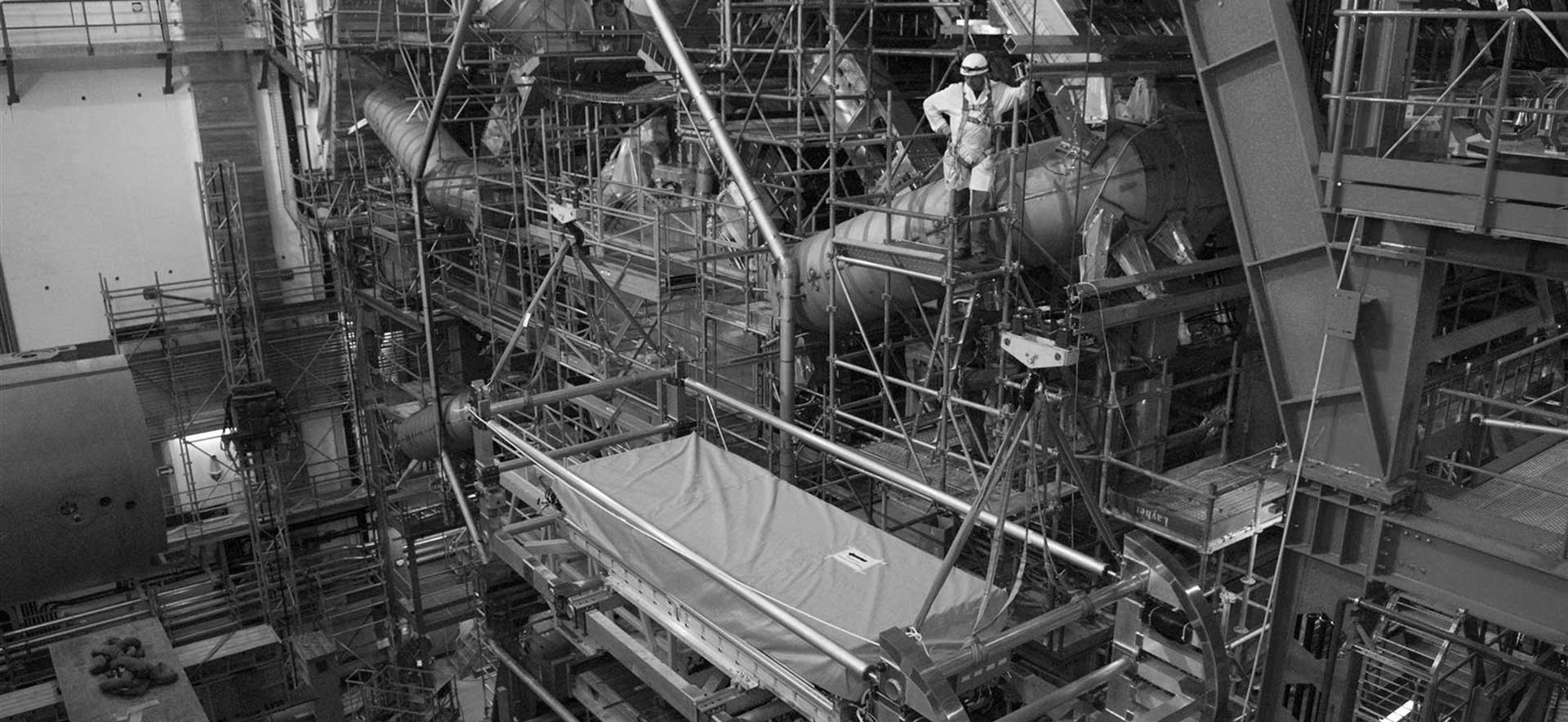
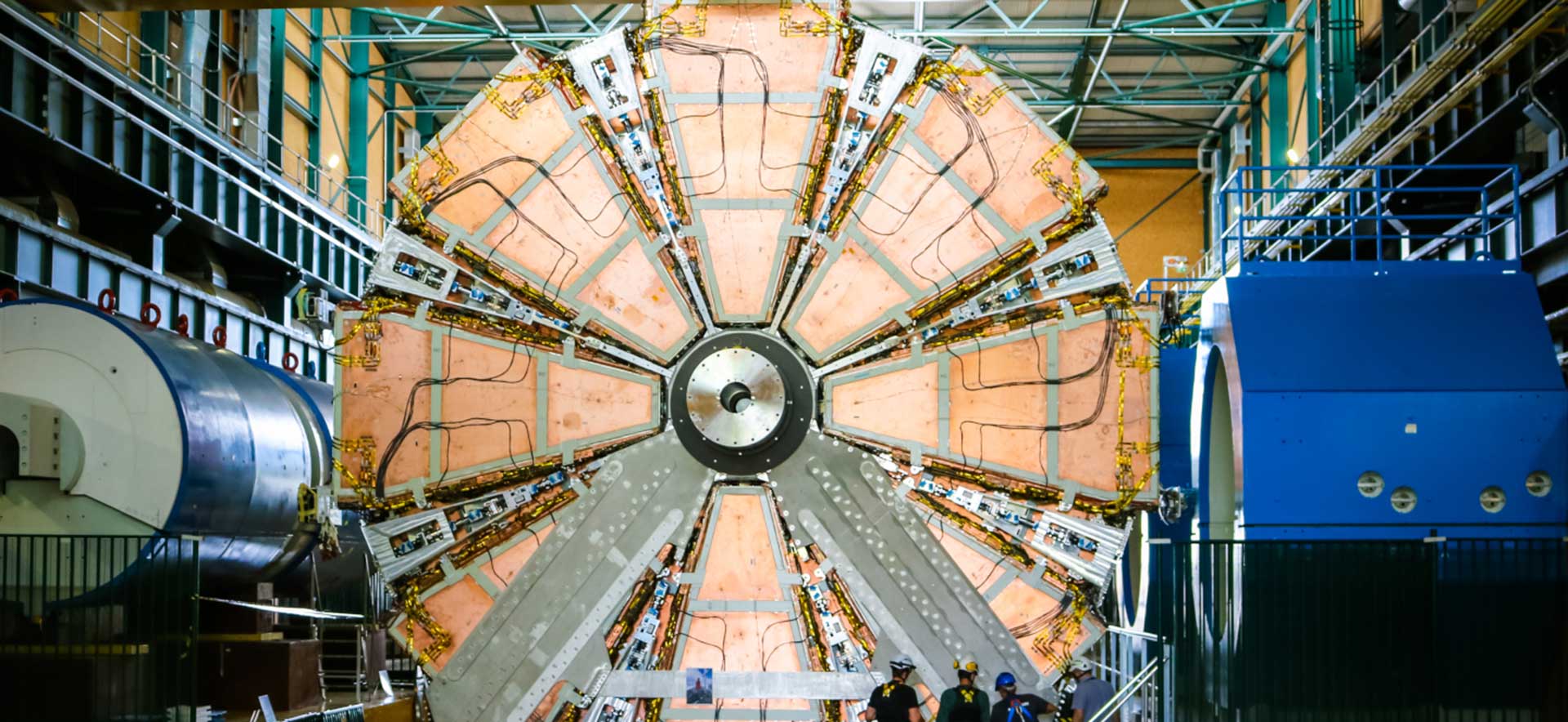
More than 300 feet underground, outside Geneva, Switzerland, trillions and trillions of subatomic particles streak around a massive man-made track at nearly the speed of light. As the particles collide and break apart, sensors record and relay a treasure trove of data to supercomputers. Physicists from around the globe study the data in pursuit of answering — and asking — fundamental questions about the universe.
This powerful particle accelerator is known as the Large Hadron Collider. It’s where some of modern physics’ most significant discoveries have been made, including the 2012 identification of the Higgs boson, the culmination of a 50-year search. The Higgs particle gives mass to all other particles. Until a decade ago, it was the missing piece of the Standard Model of particle physics, currently the best theory explaining the forces locked inside infinitesimal specks of matter and the laws governing that matter, the building block of everything from humans to galaxies.
Brandeis’ high-energy physics group played a leading role in developing the technology used to discover and research the Higgs over the past decade. Now the collider is set to be upgraded to make still more revolutionary discoveries, and a new generation of Brandeis physicists are poised to lead in the exploration of the universe.
Then: A search for the long-elusive Higgs
James Bensinger
In 1993, the U.S. Congress canceled funding for a supercollider already under construction in Texas in favor of funding the International Space Station. The collider project had been a collaboration between the federal government and the country’s top high-energy physicists, including Brandeis professor James Bensinger.
“When the Texas collider went belly up, the obvious thing was to look to Europe,” Bensinger recalls.
Across the Atlantic, in Switzerland, physicists were collaborating on plans for the LHC at the European Organization for Nuclear Research, known as CERN. The collider would set the stage for testing the theories of particle physics and search for the long-elusive Higgs in the global collaborations known as the ATLAS and CMS experiments.
Brandeis physicists, along with high-energy physicists from Harvard, MIT, Tufts, and Boston University who were part of the Boston Muon Consortium, soon decided to join their European counterparts. “If you look at who the other major contributors were when we joined, almost all of them were huge groups coming from substantial physics departments,” Bensinger recalls. “We were by far the smallest. We competed with them, and in some cases we did better.”
The LHC began operating in 2009, and on July 4, 2012, CERN announced the ATLAS and CMS experiments had finally discovered the Higgs boson particle.
The Brandeis team delivered a key contribution to this major discovery. Bensinger and colleagues Hermann Wellenstein and Craig Blocker had spent decades designing, building, and operating a detector for muons, subatomic particles produced in collisions at the LHC and key tools in identifying the Higgs. Making the detector work required virtual perfection. “The muon particles have a 14-meter pathway, and the deviation is very small,” Bensinger says. “To find out what we wanted to find out, we had to measure muon trajectories to an accuracy of the width of a human hair.”
The muon detector is still in use today.
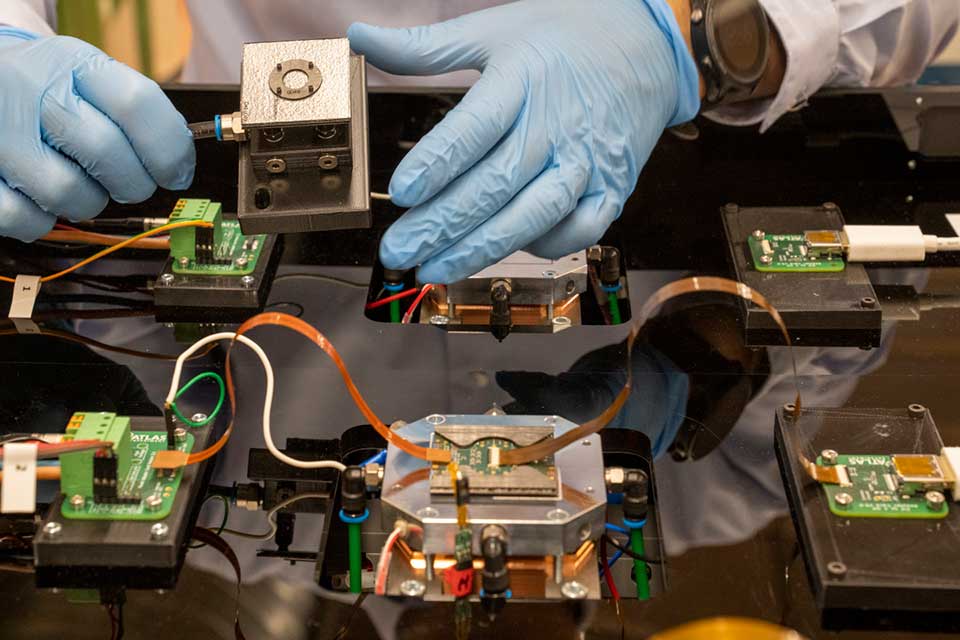
The ATLAS experiment is planning a complete replacement of its inner detector with a new all-silicon inner tracker for the high luminosity phase of the LHC. The new detector is designed to cope with the increased pile-up, data rates and radiation levels, while maintaining or improving the current tracking performance.
Photo Credit: Cavazza, Marina. Courtesy, CERN
Now: The mysteries of dark matter
While the Higgs discovery solved a long mystery, it also opened the door to numerous new, fundamental questions to explore.
Gabriella Sciolla
“Understanding what the Higgs is and testing it for the first time against the predictions of the theory is just as important as discovering the particle,” says Gabriella Sciolla, who joined the Brandeis physics group in 2011.
Exploring the behavior of the Higgs may even hold the answer to one of the universe’s greatest mysteries: dark matter. While the Standard Model offers an explanation for particles that can be detected by light, these particles account for only about 15% of the universe’s mass. The rest is dark matter, mysterious and difficult to detect — the frontier of physics.
“If we were able to observe interactions of Higgs with dark matter, that would be a revolution in particle physics,” Sciolla says. “It would be the beginning of a whole new theory.”
Bensinger and Blocker are now professors emeriti, and Wellenstein died in 2019 at age 78. But Brandeis’ work at CERN is far from over.
“If we were able to observe interactions of Higgs with dark matter, that would be a revolution in particle physics. It would be the beginning of a whole new theory.”
Gabriella Sciolla
Sciolla was new to Brandeis and the ATLAS experiment when the Higgs was discovered. She came from MIT, where she had run her own research group and co-founded a collaboration to develop a novel directional detector for dark matter. The chance to collaborate at CERN was an exciting opportunity, she says.
“It’s the only place on Earth where we can produce a Higgs boson,” Sciolla says. “No one else can. No other accelerator, no other lab can produce Higgs bosons now or in the near future. There is no other approved experiment for this.”
Aram Apyan
Aram Apyan joined the Brandeis high-energy physics group in 2021 as an assistant professor. His research focuses on studying the properties of the Higgs boson and searching for “new physics” — evidence of theories beyond the Standard Model.
"We have new data coming from the LHC right now,” Apyan says. “That gives us the opportunity to test the Higgs boson properties with unprecedented precision and to ask new questions on a larger data set. Perhaps we’ll find something new and unexpected. We are really trying to push the envelope of how much data we can collect with our detector.”
CERN is preparing to upgrade the LHC to increase its luminosity by a factor of 10, enabling more frequent — and powerful — collisions to occur. The upgrade also requires new, more durable, and more sensitive detectors. Sciolla is leading the way on their construction.
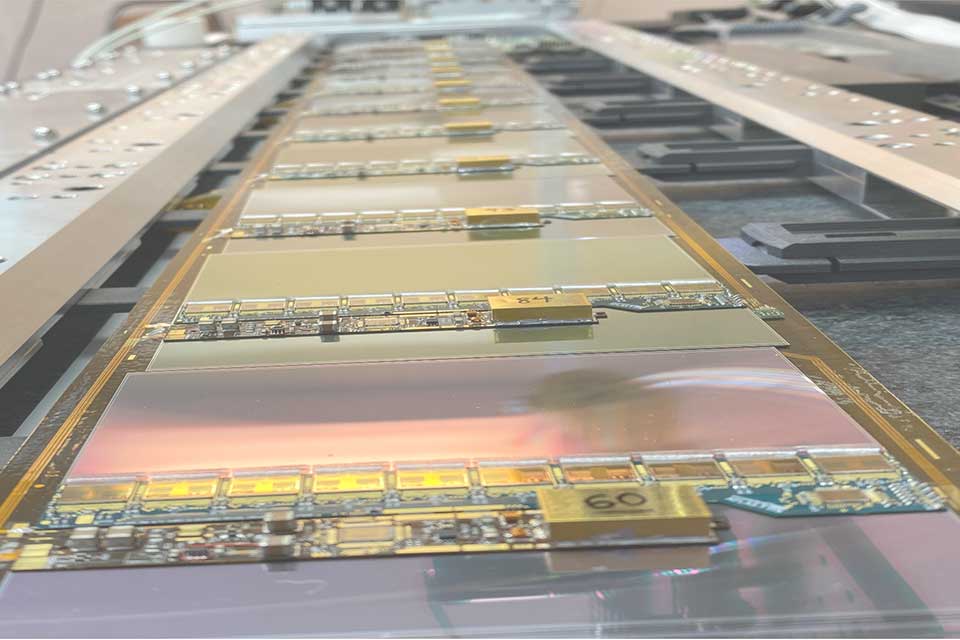
Components of the all-silicon inner tracking detector at Brookhaven National Laboratory.
Photo Credit: Anthony, Katarina. Courtesy, CERN
Next: Building a more sensitive detector
At Brookhaven National Laboratory, a U.S. Department of Energy facility on Long Island, New York, Sciolla is the U.S. coordinator for the creation of an all-silicon inner tracking detector for the ATLAS experiment. As was true for the sensor built by Brandeis physicists a generation ago, precision is key.
“Every single chip we use has to be custom-designed, and they all have to be extremely fast, because they are going to take huge amounts of data per second,” Sciolla says.
The collider upgrades, which should be finished by 2029, will improve precision by a factor of five, and could make possible the observation of never-before captured self-interactions of the Higgs.
Along with Sciolla and Apyan, dozens of Brandeis alumni and students are contributing.
One such contributor, Hannah Herde, GSAS PhD’20, an associate senior lecturer at Sweden’s Lund University, specializes in the construction and design of particle detectors used at the LHC. In 2010, when she was a Wellesley College undergraduate majoring in physics and classics, she attended a colloquium delivered by guest lecturer Gabriella Sciolla.
“Gabriella laid out, over the course of an hour, how the collisions collected with ATLAS are pieces of a jigsaw puzzle. When the pieces come together, they show what the fundamental building blocks of nature are doing,” Herde says. “I thought it was extraordinary. Later on, we got to talking. Eventually, it became clear that studying physics at Brandeis would be an incredible opportunity."
As a PhD candidate, Herde was part of the original Brandeis team to work at Brookhaven National Lab, an opportunity that shaped her professional path.
“Gabriella’s passion for physics and her mentorship led me to become a particle physicist,” Herde says. “She’s big on having a plan. The plan can and should change over time, but you should have some guiding principles, and you can see she follows that approach in her own research and in how she mentors students.”
Today, a new crew of Brandeis graduate students work at Brookhaven, on campus in Waltham, and at the Large Hadron Collider. Emily Duden, a second-year graduate student, spent last summer working at Brookhaven and analyzed particle data on campus during the school year.
“There’s no next generation of particle physics without a next-generation particle detector,” Duden says. “As a young student at a really pivotal time in the field, I’m doing work that really positions me to be a leader in the field later in my career.”
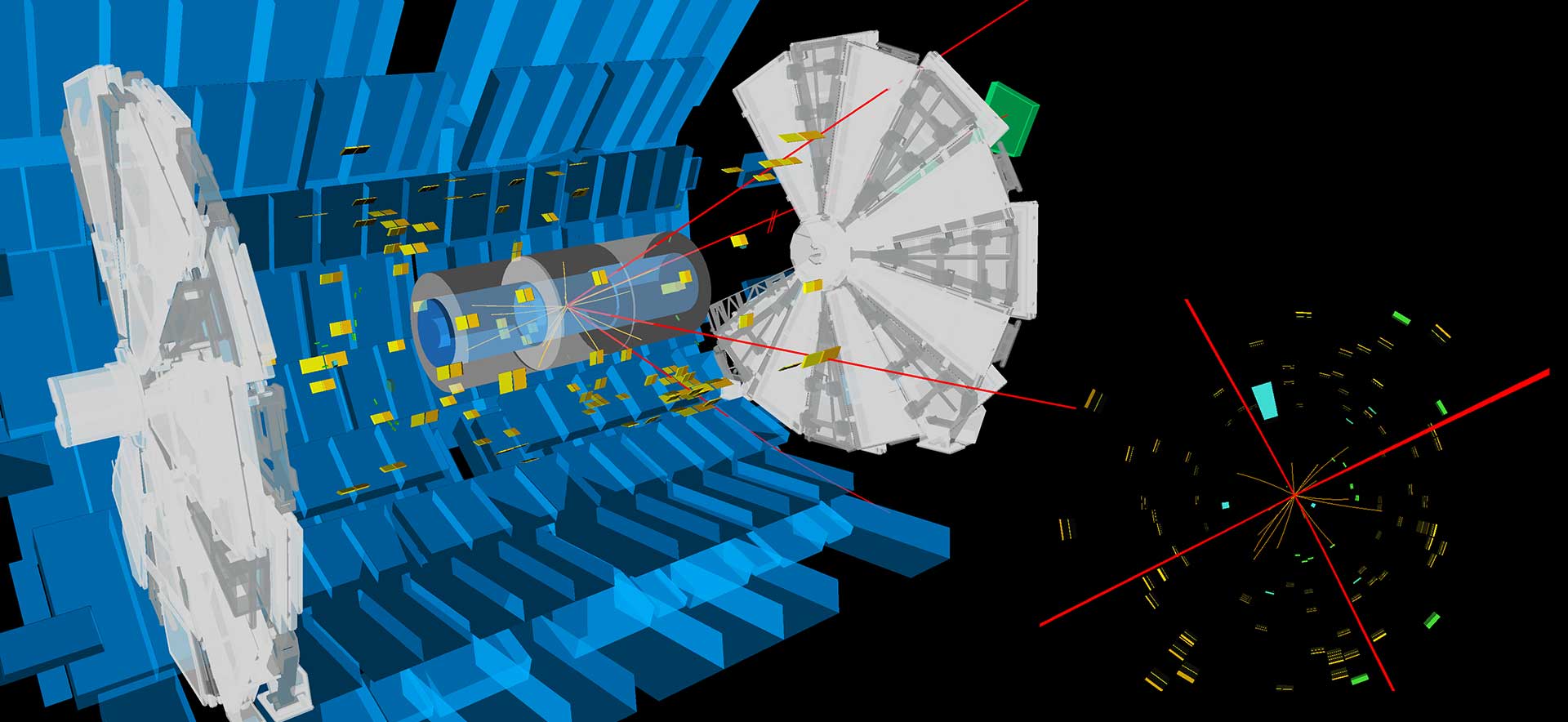
A Higgs boson candidate decay to four muons (H → ZZ* → 4μ). The two muons are highlighted in red. The measured four lepton invariant mass is 125.5 GeV.