IRG 1: Self-Limiting Assembly
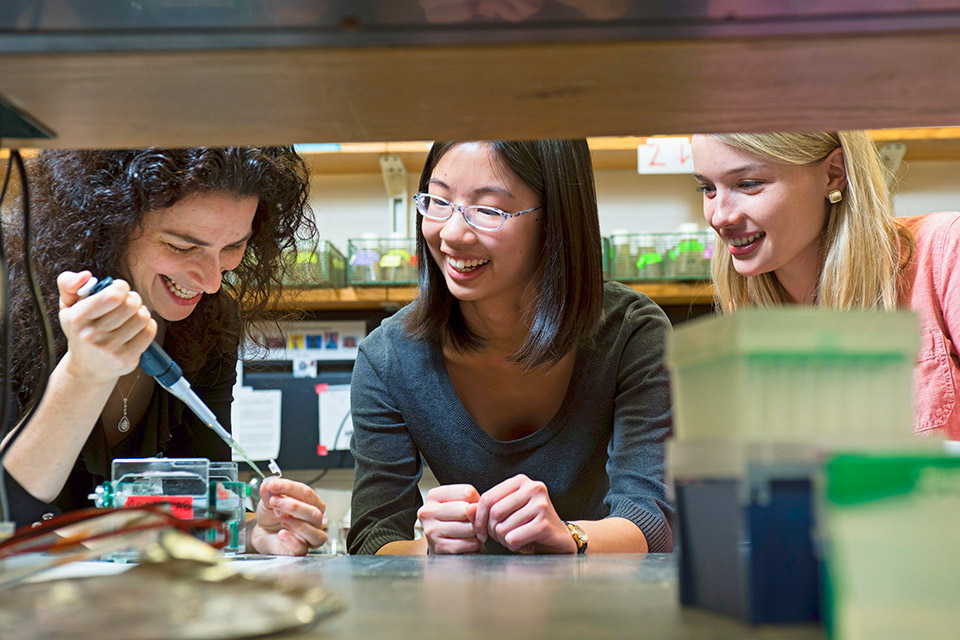
Research Vision and Plan
Self-assembly of complex and functional materials into large, but finite-size, superstructures remains a grand challenge in soft materials science. Living systems routinely achieve size-controlled assembly, such as bacterial microcompartments like carboxysomes, and fibers with finite diameters like collagen. In contrast, most inorganic materials form unlimited structures like crystals, and synthetic approaches to size-controlled assembly lag far behind biology. Thus, learning to achieve robust, self-limited assembly is essential to understand the Rules of Life. Further, learning to engineer the self-assembly of synthetic self-limiting structures would enable scalable design of new functional and adaptable materials, such as paintable photonic coatings, injectable biomedical scaffolds, and capsids for drug and gene therapy.
Inspired by biology, IRG1 will develop a suite of synthetic building blocks that, without requiring external control, undergo equilibrium self-assembly that terminates at a prescribed finite-size. Self-limiting assembly will be enabled by using DNA origami and polymeric gel particles to create building blocks with programmable shape, anisotropic interactions, and flexibility. These blocks far surpass current “patchy” particles in the ability to design lock-and-key interactions with kBT-scale precision of affinities and nm-scale control over directionality. Further, our approach transcends the concept of patchy particles by designing the deformability of building blocks to control assembly.
IRG 1 will pioneer new routes to engineer self-regulating materials with tunable sizes through assembly of programmable building blocks. We will advance two paradigms: Thrust 1: Curvature-Controlled Assembly, using “tapered monomers” that form self-closing objects, and Thrust 2: Frustration-Controlled Assembly, using “frustrated monomers” that form self-limiting structures by distorting and accumulating strain upon assembly. Both thrusts seek robustly self-regulating, equilibrium mechanisms that autonomously terminate at programmable finite sizes, without the external environmental control required by current methodologies.
To meet this challenge, IRG1 will take a closed-loop data-driven approach enabled by a multiscale theoretical, computational, and experimental framework. High-resolution cryo-EM of the blocks and designed assemblies, combined with measurements of assembly kinetics, will provide data to iteratively redesign the building blocks and refine the assembly models.
Primary Participants
-
Mike Hagan, (group co-leader), Brandeis Physics
- Ben Rogers, (group co-leader), Brandeis Physics
-
Greg Grason, UMass Amherst, Polymer Science and Engineering
-
Seth Fraden, Brandeis Physics
-
Tijana Ivanovic, Brandeis Biochemistry
-
Ryan Hayward, University of Colorado Boulder, Polymer Science and Engineering
-
Avi Rodal, Brandeis University Biology
-
Bing Xu, Brandeis Chemistry
Primary National Labs and/or International Participants
-
Hendrick Dietz, TU Munich
Secondary Participants
- Jonathan Doye, University of Oxford Theoretical Chemistry
- Chris Santangelo, Syracuse University
- Mark Stevens, Sandia National Labs
IRG1 Seed
- Grace Han, Brandeis Chemistry